E-Archive
Science Update
in Vol. 5 - May Issue - Year 2004
Fatigue Response of the Various Titanium Alloy Classes to Shot Peening
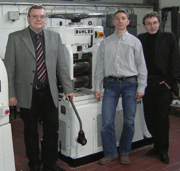
From left to right: Prof. L. Wagner, Dipl.-Ing. T. Ludian and Dipl.-Ing. M. Kocan
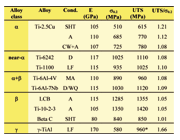
* in compression Table 1. Tensile Properties of the various titanium alloys
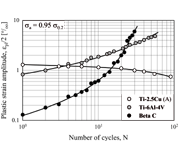
a) Various titanium alloys
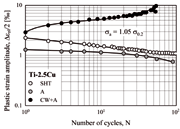
b) Ti-2.5Cu in various conditions Figure 1. Cyclic deformation behvior
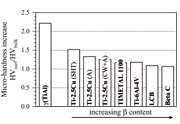
Figure 2. Surface microhardness changes after shot peening
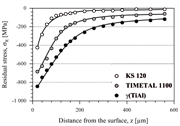
Figure 3. Residual stress-depth profiles after shot peening
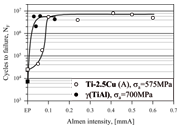
a) a alloy and y titaniumaluminide

b) (a+B) alloys

c) metastable B alloys Figure 4. Fatigue life vs. Almen intensity
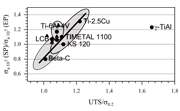
Fig. 5. HCF strength increases vs. work-hardening capability
Prof. Lothar Wagner (Ph.D), Dipl.-Ing. Marcin Kocan and Dipl.-Ing. Tomasz Ludian, Institute of Materials Science and Technology Technical University of Clausthal, Germany
Abstract
Potential improvements in fatigue performance of the various titanium alloy classes by shot peening are outlined. Alloys covered belong to , near-?, ?+?, and metastable ? alloy classes. In addition, results on a titanium aluminide ?(TiAl) are also presented. After shot peening, marked differences were found in the process-induced microhardness depth profiles among the various alloys which correlated with the work-hardening capability in monotonic loading. Most pronounced improvements in HCF life were observed on an a titanium alloy and a titanium aluminide while only slight improvements were found on metastable alloys. Near-? and ?+? alloys showed an intermediate response. The fatigue results are interpreted with the cyclic stability of the process-induced changes in microstructure and residual compressive stresses.
Introduction
The marked improvement of the HCF performance of light alloys based on titanium, aluminum and magnesium [1, 2] is mainly attributed to process-induced changes in near-surface microstructure, e.g., increases in dislocation densities and the development of residual compressive stresses.
The increase in dislocation densities led to higher resistances to fatigue crack nucleation while residual compressive stresses are known to hinder or even stop micro-crack growth [3]. Work on metastable titanium alloys such as Beta C, Ti-10-2-3 and LCB [4-6] showed that their fatigue response to shot peening was significantly less beneficial than found in earlier work on ?+? titanium alloys such as Ti-6Al-4V [3]. The present investigation is aimed at understanding the different fatigue response of the various titanium alloy classes to shot peening.
Experimental
Alloys studied included Ti-2.5Cu of the titanium alloy class, Ti-6242 and TIMETAL 1100 representing near-? alloys, Ti-6Al-4V and Ti-6Al-7Nb as ?+? alloys as well as Ti-10-2-3, LCB and Beta C belonging to the metastable ? alloy system. In addition, the intermetallic compound (TiAl) was included in this study.
Ti-2.5Cu was tested in the following conditions: solution heat treated (SHT), conventionally two-step aged (A) and cold worked plus aged (CW+A). The near- alloys Ti-6242 and TIMETAL 1100 were prepared with duplex and fine-grained fully lamellar microstructure, respectively. The ?+? alloys Ti-6Al-4V and Ti-6Al-7Nb were investigated having mill annealed and duplex microstructures, respectively. Of the metastable ? alloys, Ti-10-2-3 and LCB were prepared to exhibit fully aged ? matrices with about 15 % primary contents. Beta C was tested in a ?-solution heat treated (SHT) condition. Details of the thermomechanical treatments utilized are described in [4-8].
Tensile test results on the various alloys and aging conditions are listed in Table 1.
To determine the cyclic deformation behavior of the various alloys which is argued to affect the cyclic stability of residual stresses, stress controlled low cycle fatigue (LCF) tests were performed on threaded cylindrical specimens having gauge lengths and diameters of 13 and 4 mm, respectively. These tests were done under axial loading at a stress ratio of R = -1 and a frequency of 0.05 Hz by means of a servohydraulic testing machine. The axial strain was recorded by strain gauges. The plastic strain at zero load was plotted versus number of cycles.
For HCF testing, hour-glass shaped specimens with a gauge diameter of 3.6 mm were machined. Part of the specimens was electro-polished (EP) to serve as a reference. 100 m were removed from the as-machined surface to ensure that any machining effect that could mask the results was absent.
The other part was shot peened (SP) by means of an injector type machine or a direct pressure blast system using various shot materials. Details are given in [7]. Peening was done to full coverage to various Almen intensities ranging from 0.05 to 0.60 mmA.
The change in surface layer properties caused by shot peening was characterized by microhardness-depth profiles and residual stress-depth-profiles measured by the incremental hole drilling method as described elsewhere [9].
HCF tests were performed in rotating beam loading (R =-1) at frequencies of about 60Hz.
Results and Discussion
Cyclic Deformation Behavior
The cyclic deformation behavior of the various alloys and aged conditions is illustrated in Fig. 1.
The a alloy Ti-2.5Cu in the conventionally aged condition shows cyclic hardening while significant cyclic softening was observed in mill annealed Ti-6Al-4V (Fig. 1a). Cyclic softening in various conditions of Ti-6Al-4V was also reported [10]. Very marked cyclic softening was found in solution heat treated Beta C (Fig. 1a). Cyclic softening was also reported in parallel work on the metastable alloys LCB [6] and Ti-10-2-3 [11].
For a given alloy, the microstructure clearly affects the cyclic deformation behavior as shown on Ti-2.5Cu in Fig. 1b. The condition CW+A cyclically softens while both conditions SHT and A cyclically harden (Fig. 1b).
Obviously, the observed ranking in cyclic deformation behavior (Fig. 1) can be correlated in a first approximation with the tensile properties of the various alloys (Table 1). Alloys which show pronounced strain-hardening (e.g., UTS/?0.2>1.1) tend to cyclically harden while alloys with low work-hardening capability (e.g., UTS/?0.21.1) tend to cyclically soften (compare Fig. 1 with Table 1).
In previous work on Ti-2.5Cu and Beta C, it was found that the character of the cyclic deformation behavior was not changed if the materials were cold worked to simulate surface layer properties after shot peening [11]. Since residual (elastic) stresses will decrease if elastic strains are converted into plastic strains during fatigue loading, it can be anticipated that alloys which cyclically harden will respond to a shot peening treatment regarding fatigue performance more beneficially than alloys which cyclically soften.
Surface Layer Properties
Comparing the micro-hardness increases from the bulk to the surface for a variety of shot peened titanium alloys and the titanium aluminide (Fig. 2), the most marked increases were found for (TiAl) followed by Ti-2.5Cu, Ti-6Al-4V and TIMETAL 1100. Low hardness increases were observed on the metastable alloys for both age-hardened LCB and solution heat treated Beta C. Apparently, there seems to exist a correlation between the amount of phase in titanium alloys and their work-hardening capability.
Typical residual stress-depth profiles as measured by the incremental hole drilling method are shown in Fig. 3.
Generally, high residual compressive stresses were measured at the surface with a gradual decrease in the direction of the interior of the materials. Interestingly, residual compressive stresses in (TiAl) can reach values much higher than the tensile yield stress of the virgin material (compare Fig. 3 with Table 2) reflecting again the enormous work-hardening capacity in this -titanium aluminide. Pronounced work-hardening of -titanium aluminides after machining was also reported in the literature [11].
HCF Performance
Fatigue Life
The effect of shot peening using a wide variation in Almen intensity on the fatigue life at given stress amplitudes is illustrated for the various titanium alloys and the (TiAl) in Fig. 4.
Obviously, Ti-2.5Cu in condition A as well as (TiAl) (Fig. 4a) respond most beneficially to shot peening. For both materials, the fatigue life for the tested stress amplitudes is increased by almost 3 orders of magnitude. An improvement by one order of magnitude was observed on the ?+? alloys Ti-6Al-4V and Ti-6Al-7Nb (Fig. 4b) whereas on average, the life improvement was even lower for the metastable alloys (Fig. 4c).
Fatigue Strength
The HCF response to shot peening of all titanium materials studied in this work is summarized in Fig. 5 where the change in the 107 cycles fatigue strengths due to shot peening is plotted vs. the work-hardening capability. Although there is some scatter, the general trend is clear. The higher the work-hardening capability of a material, the more beneficial will be its HCF response to shot peening.
Summary
The results of this investigation indicate that the fatigue response of titanium alloys and titanium aluminides to mechanical surface treatments depends on their work-hardening capability and cyclic deformation behavior. Severe cyclic softening in metastable ? alloys leads to only small improvements or even to a deterioration of the HCF strength after shot peening while ?+? and near-? alloys that only slightly cyclically soften respond more beneficially. Pronounced cyclic hardening in ?(TiAl) and the alloys lead to the most marked HCF improvements in life. Presumably, the cyclic deformation behavior affects the cyclic stability of the process-induced changes in the near surface regions of the materials, namely dislocation strengthening and residual compressive stress fields.
Acknowledgements
Most of the reported work was performed at the Technical University of Brandenburg at Cottbus. The authors would like to thank their former coworkers Drs. A. Berg, T. Dörr and A. Drechsler and J. Lindemann for their contributions. Financial support by the Deutsche Forschungs-gemeinschaft (DFG) over the last decade is gratefully acknowledged.
References
1. L. Wagner, Materials Science and Engineering A 263, 1999, p. 210.
2. I. Altenberger, S. Jägg, B. Scholtes, Werkstoffprüfung 98, DVM, 1998, p. 55.
3. L. Wagner, G. Lütjering in Shot Peening (Niku-Lari, ed.), Pergamon Press, 1982, p. 453.
4. A. Drechsler, T. Dörr, L. Wagner, Materials Science and Engineering A, 1998, p. 146.
5. L. Wagner, A. Berg, T. Dörr, M. Hilpert, Mechanische Oberflächenbehandlungen (Eds: H. Wohlfahrt and P. Krull) Wiley-VCH, 2000, p. 179.
6. J. Kiese, J. Zhang, O. Schauerte, L. Wagner in Shot Peening (L. Wagner, ed.) Wiley-VCH, 2003, p. 380.
7. J. K. Gregory, L. Wagner in Shot Peening (L. Wagner, ed.) Wiley-VCH, 2003, p. 349.
8. J. Kiese, L. Wagner, J. K. Gregory, 4th European Conference on Advanced Materials and Processes, FEMS, 1995.
9. J. Lindemann, D. Roth-Fagaraseanu, L. Wagner in Light Materials for Transportation Systems (N. J. Kim, C. S. Lee, D. Eylon, eds), Center for Advanced Aerospace Materials, 2001, p. 793.
10. M. A. Däubler, PhD-thesis, Ruhr-University Bochum, 1980.
11. P. E. Jones, D. Eylon, Titanium ’98, Proceedings of Xi’an International Titanium Conference (L. Zhou, D. Eylon, G. Lütjering, C. Ouchi, eds.), International Academic Publishers, 1999, p. 113.
For Information:
Institute of Materials Science and
Engineering, TU Clausthal
Agricolastrasse 6
38678 Clausthal-Zellerfeld, Germany
Tel.: +49.5323.72 2770
Fax: +49.5323.72 2766
Email: lothar.wagner@tu-clausthal.de
