E-Archive
Science Update
in Vol. 16 - January Issue - Year 2015
Finishing of Plastics
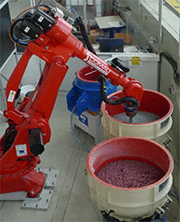
Figure 1: Robot-guided drag finishing at Production Technology Center (PTZ) Berlin
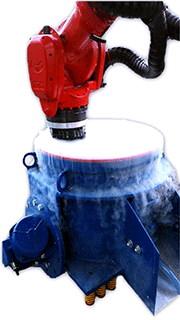
Figure 2: Cooling media by adding dry ice
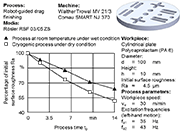
Figure 3: Resulting percentage of initial surface roughness Ra
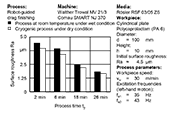
Figure 4: Resulting surface roughness Ra for selected process times
Introduction
Mass finishing is used for a wide range of deburring and polishing tasks. Conventional mass finishing processes like tumble, centrifugal disk and vibratory finishing take advantage of large batch sizes, as a bulk of workpieces can be finished simultaneously. As a major disadvantage of these processes, workpieces can collide at any time. This is especially undesirable for complex or functionally critical workpieces, for example, medical and aircraft components. To overcome this difficulty, a new process called robot-guided drag finishing has been developed and patented at the Production Technology Center (PTZ) Berlin [UHL11, UHL14], Figure 1. In this process, a Comau NJ 370 6-axis industrial robot drags workpieces through abrasive media that can be agitated by a vibratory finishing bowl. The setup allows for outstanding kinematic flexibility in terms of the workpiece path, thus enhancing the fields of application of mass finishing.
During the last few years, an increasing number of companies was challenged with tasks in finishing plastics and fiber-reinforced plastics to a very fine surface roughness. In contrast to conventional metal machining, finishing of these materials should mostly be done without lubrication in a dry process avoiding material damage by water. As major drivers of innovation in German mass finishing research, scientists at the Production Technology Center (PTZ) Berlin and at the Laboratory for Machine Tools and Production Engineering (WZL) Aachen teamed up to take up this challenge and present first results in this science update.
State of the art
Conventional mass finishing is used for metallic, plastic and ceramic workpieces for deburring, cleaning and edge rounding. In general, the improvement of the workpieces' surface is the central assignment of mass finishing [KLO05]. An essential influence on the surface quality of mass finished workpieces is the velocity of the workpiece in relation to the abrasive media. In the robot-guided drag finishing setup, high velocities and kinematic flexibility can help to decrease process times.
In mass finishing, lubrication by a water-compound mixture is typically used to clean workpieces and media, remove scale, and inhibit corrosion of metal workpieces. Furthermore, it has been shown that roughness changes are strongly dependent on lubrication [WAN00, BAG03]. It can be assumed that this is caused by the absence of media sliding on workpieces' surfaces in dry condition, as YABUKI ET AL. [YAB02] have observed. This observation was also verified for robot-guided drag finishing at the Production Technology Center (PTZ) Berlin by tracking media movement with a camera system that is currently developed at Laboratory for Machine Tools and Production Engineering (WZL) of RWTH Aachen University. Compared to processes under wet conditions, the absence of sliding causes impacts that are of higher force and more frequent with orientation mostly in normal direction to workpieces' surfaces [YAB02].
Robot-guided drag finishing of plastics
Some materials like plastics and fiber-reinforced plastics should not be finished under wet conditions because the absorption of water can affect the mechanical properties [LEC10]. Both material groups are increasingly used in industrial applications. Consequently, process design of robot-guided drag finishing of plastics and fiber-reinforced plastics under dry condition is of high interest for both scientific and the industrial sections.
For the investigations presented in this paper, two plastics, namely ultra-high molecular weight polyethylene (PE-UHMW) and polycaprolactam (PA 6), which are widely used thermoplastics, are considered. In a subsequent study, workpieces made of carbon and glass fiber-reinforced plastics are to be investigated. The workpieces, used here, were cylindrical plates with a diameter of 100 mm and a thickness of 10 mm that were finished for 60 minutes in total. The lateral surface of the workpieces was turned beforehand with defined parameters so that their initial surface is of a comparable roughness. After each process step, surface roughness is measured at six evenly spread positions on the lateral surface. As abrasive media, RSF 03/05 ZS (cylindrical shape angle cut, ceramic bond) and RKV 12K (cone shape, plastic bond) are used. Moreover, the experiments were carried out under both wet and dry conditions and the used velocities were 10 m/min and 30 m/min respectively.
The best surface roughness in robot-guided drag finishing under dry conditions was measured using the abrasive media RSF 03/05 ZS and a workpiece speed of 30 m/min. After 60 minutes, a reduction of surface roughness Ra to 23 % of the initial value was measured for PE-UHMW. Using a low workpiece speed of 10 m/min, best surface roughness under dry conditions is achieved with PE-UHMW using abrasive media RSF 03/05 ZS resulting in a reduction of surface roughness Ra to 29 % of the initial value. Hence, the influence of the workpiece speed on roughness that is known for metal finishing, can be confirmed for plastics. Basically, reachable roughness under dry conditions is worse than under wet conditions. This is especially obvious for PA 6 material and a workpiece speed of 10 m/min with a reduction of surface roughness Ra to 29 % of the initial roughness under wet and 41 % of the initial roughness under dry conditions. This finding conflicts with the expected increasing material removal rate because of higher forces at workpiece-media contacts in dry conditions, as described by YABUKI ET AL. [YAB02]. A possible explanation is that plastic material tends to retreat reversibly under loading, which is an elastic deformation that does not imply an improvement in surface roughness.
It is also noted, that compared to reachable roughness on PE-UHMW workpieces, it gets worse for PA 6 under both wet and dry conditions. The difference in ball indentation hardness is supposed to be accountable for this discrepancy, as PA 6 has a ball indentation hardness of 170 N/mm2 and PE-UHMW of 35 N/mm2 respectively. It is supposed that a lower hardness can lead to better roughness after mass finishing.
Development of a cryogenic robot-guided drag finishing process
Changing the workpiece material characteristics by cooling either workpiece or media below room temperature seems to be a feasible way of improving the process. Consequently, a cryogenic process was designed. This process aims at higher material removal rates due to the brittleness of materials at low temperatures. As a consequence, it is expected that the impact force needed for plastic deformation is decreased and hence roughness can be improved further. To check whether such an embrittlement can be reached both ways, direct cooling of workpieces or cooling of media were investigated. Workpiece temperature was acquired with a 0.25 mm K-type thermocouple that fixed in the workpiece.
For cooling media, dry ice pellets (diameter 3 mm, length 5 mm) were scattered in the bulk of media (Figure 2). This leads to a workpiece temperature of about 10°C.
To further decrease the workpiece temperatures, liquid nitrogen was used to directly cool workpieces before the process is started or continued (so-called cryogenic processing). With direct cryogenic cooling, workpiece temperatures of -140°C were reached. Following, the results of cryogenic mass finishing under dry conditions are presented and compared to conventional mass finishing under wet conditions.
Comparison of the results
The development of surface roughness Ra over process time in the cryogenic process shows a clear difference compared to conventional mass finishing under wet conditions (Figure 3). The surface roughness Ra in a wet process is reduced to 64 % of the initial roughness after 14 minutes of mass finishing. In contrast, surface roughness decreases faster in a cryogenic process and reaches a value of 52 % of the initial roughness after the same process time.
Looking at the absolute values of the surface roughness Ra, Figure 4 reveals similar findings. The cryogenic process leads to the best results for the two different processes at every process step. Even after short process times, the resulting surface roughness (3.7 µm) is better than in the wet process (4.4 µm). Finally, after 26 minutes of cryogenic processing, a surface roughness Ra = 1.4 µm is achieved. Thus, surface roughness is less than one-third of the initial value due to cryogenic process!
Summary and outlook
It is shown that processing of plastics under dry conditions is possible at the cost of worse surface roughness in comparison to processes with lubrication. To overcome this challenge, a cryogenic process using liquid nitrogen as coolant has been developed. On the basis of the presented finishing strategies under dry or even cryogenic conditions, new applications of mass finishing will be available. Especially with regard to the cryogenic processing, finishing of elastomers is just around the corner. It should also be checked whether the presented perceptions could be transferred to mass finishing of other materials, like metals and fiber-reinforced plastics. To increase the identified benefit in roughness change by embrittlement, currently further cooling concepts are being evaluated at the Production Technology Center (PTZ) Berlin that enable a permanent in situ direct cooling of workpieces.
References
[BAG03] Baghbanan, M.R.; Yabuki, A.; Timsit, R.S.; Spelt, J.K.: Tribological behavior of aluminum alloys in a vibratory finishing process. Wear 255 (2003) 7 - 12, p. 1369 - 1379.
[KLO05] Klocke, F.; König, W.: Fertigungsverfahren 2. Schleifen, Honen, Läppen. 4. Aufl. Berlin: Springer, 2005. P. 427.
[LEC10] Lechner, M. D.; Gehrke, K.; Nordmeier, E. H.: Makromolekulare Chemie. Ein Lehrbuch für Chemiker, Physiker, Materialwissenschaftler und Verfahrenstechniker. 4. Aufl. Basel: Birkhäuser, 2010. P. 496.
[UHL11] Uhlmann, E.; Dethlefs, A.: Polieren komplexer Bauteile. In: WB Werkstatt + Betrieb. 144. Jg., 2011, Nr. 6, S. 28-31.
[UHL14] Uhlmann, E.; Dethlefs, A.: Robot-Guided Finishing. Current Research Topics. In: MFN Metal Finishing News. 15. Jg., 2014, Nr. 1, pp. 58-59.
[WAN00] Wang, S.; Timsit, R. S.; Spelt, J. K.: Experimental investigation of vibratory finishing of aluminum. In: Wear 49. Jg., 2000, Nr. 243, pp. 147-156.
[YAB02] Yabuki, A.; Baghbanan, M.R.; Spelt, J.K.: Contact forces and mechanisms in a vibratory finisher. In: Wear. 51. Jg., 2002, Nr. 252, pp. 635-643.
For Information:
M. Sc. Alexander Eulitz, Research Engineer
Institute for Machine Tools and Factory Management, Technische Universität Berlin
Tel: +49.30.314 24963
E-mail: eulitz@iwf.tu-berlin.de
1Institute for Machine Tools and Factory
Management, Technische Universität Berlin
2Laboratory for Machine Tools and Production Engineering (WZL) Aachen
